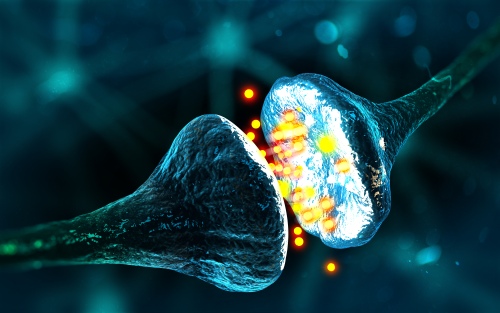
Seventy years ago, a chemist by the name of Eugene Roberts was meticulously analyzing amines from brain extracts, when he came across one that did not match any previously known compound. Not only this, but this particular molecule also seemed significantly concentrated in the brain compared to any other tissue. In an era of no mass spectrometry or other tools we have come to consider standard, further analysis showed this peculiar substance was γ-amino butyric acid.1,2
Far from immediately igniting a revolution in neuroscience, it wasn’t until seven years later that this discovery came to bear functional meaning. It was a known fact that mammalian brain extracts can exert an inhibitory activity on an isolated crayfish neuron. The elusive ‘factor I’, as the agent responsible for this effect was initially coined, proved to be none other than γ-amino butyric acid.1,3 Today we refer to it, in short, as GABA.
How GABA works
GABA is the primary neurotransmitter employed by inhibitory neurons, both in the spinal cord and the brain. Otherwise distinct types of neurons – such as medium spiny neurons in the striatum, basket cells in the cerebellum and hippocampus, Purkinje cells in the cerebellum - work in a remarkably uniform manner. Upon stimulation, they produce GABA and release it within the synaptic cleft.4

GABA then binds to its specific receptor and inhibits the downstream neuron. What this highly-studied ‘inhibition’ actually means, for the neuron, is that it becomes much more difficult for it to be stimulated. How that happens comes down to the complicated biophysical processes taking place on the neuron’s membrane. We’ll maybe dive into those intricacies another time. For now, it is important to know that negative charges, such as chlorine, coming into the neuron inhibit it.
This is precisely what the main GABA receptor is, in essence: a chlorine channel. When GABA binds to its specific spot, it opens the channel, and chlorine quickly flows into the neuron, causing inhibition. It is worth noting that there are, in fact, several types of GABA receptors. The one we described, termed GABA-A, is the most abundant and the one we can target with most available drugs. Other subtypes that work in slightly different manners, although still with an inhibitory outcome, are GABA-B and GABA-C. 5
What GABA does
Let’s stray away from these sub-microscopic details for a second and watch the mechanisms they produce at play. We know that upon lightly hitting the spot just below the knee, our foot jerks up due to the knee-jerk reflex. This works through a relatively simple reflex loop: The sensory neuron innervating the spot below the knee excites the neuron innervating the quadriceps muscle (the one that makes the foot jerk up). However, this loop does not act alone. The sensory neuron also excites an inhibitory interneuron, which, in turn, inhibits a motor neuron innervating the muscles on the back of the thigh – thus relaxing them, and allowing for the reflex to take place.4

Although this may be the most straightforward example of its action, there are numerous other more fascinating roles GABA serves. Many were discovered in cases where it was, in one way or another, deficient.
Since it is so widely available in the brain and able to inhibit neural circuits, a GABA deficit has long been thought to be associated with epilepsy. Furthermore, many drugs that are able to induce seizures appear to do so by inhibiting its synthesis. Thus, the theory emerged that genetic epilepsy should be linked to the GABA gene, acquired epilepsy must arise through some other form of GABA deficit, and ultimately all could be solved by drugs that properly activate the GABA receptors.
However, this GABA-ergic theory was rendered incomplete as far back as the ‘90s 6, and we understand today that it only plays a part in the complex pathology that is epilepsy.7 Nevertheless, some anticonvulsant medications available today rely on the GABA system. Vigabatrine8 and Valproic acid9, for example, are thought to inhibit GABA degradation, thus increasing its availability at the synapses.
Another logical association links GABA and anxiety. Anxiety is thought to arise from an imbalanced response to threatening stimuli. On that note, GABA is believed to play an essential role in modulating circuits associated with fear in the amygdala. A lack of appropriate GABA stimulation may then lead to a pathological anxiety state. This theory is further sustained by how effective modulators of the GABA-A receptor, such as benzodiazepines, barbiturates or even alcohol are at releasing anxiety.10
A different physiological situation in which we would need our brain to be slightly inhibited is during sleep. It is a known fact that activating GABA-A receptors (by, say hypnotics) favors sleep – falling asleep and the first, dreamless stages of it (non-REM). Antagonizing the GABA receptors, on the other hand, specifically GABA-B and GABA-C, favors REM sleep and staying awake - which, in terms of EEG activity, are quite similar.11 A recent study even describes that directly activating an area of GABAergic neurons within the brainstem induced long-lasting non-REM sleep, while damaging the same area increased wakefulness.12
GABA imbalances are even theorized to play a role in aging. The GABA system does, indeed, seem to be altered with time, either in terms of GABA synthesis, its release, or the overall inhibition it is supposed to produce.14 Recent theories propose these disturbances may play a role in the loss of movement control. Impaired modulation of the excitability along the tract that connects the cortex to our muscles may be the one responsible for the lack of coordination acquired with age.13
It is worth mentioning that all these states are by no means exclusively driven by GABA, as the neurochemical landscape of any circumstance is often complicated and involves many systems at a time. GABA does, however, play an undeniable role in the proper functioning of our nervous system, and these are just highlighting its mechanisms at play.
GABA beyond the synapse
GABA does not only act at synapses. Receptors were found to be widely distributed not only at the synaptic cleft but on the body, axon, and dendrites of neurons. Additionally, it has been proven that GABA is present at a very low concentration in the extracellular space. This means it can act on those extra-synaptic GABA receptors, producing a large scale basal level of inhibition – the so-termed tonic inhibition, which is supposed to globally modulate the excitability of a particular population of neurons.15
The number and configuration of these extra-synaptic GABA receptors vary across brain regions and cell types, which makes tonic inhibition an unequally distributed phenomenon. Areas such as the hypothalamus, the cerebellum, the striatum seem to be particularly influenced by it. Surprisingly, the level of inhibition even varies across different layers of the neocortex, different regions of the amygdala or different nuclei of the thalamus – further accentuating just how heterogeneous its effects are. 16
So, what does tonic inhibition do, or what happens when there is not enough of it: In short, epilepsy. When the excitability of a neural circuit is not modulated correctly, it can begin firing uncontrollably and cause a seizure. Consequentially: genetic anomalies of the extra-synaptic GABA-A receptors were associated with childhood epilepsy, mice models of receptor deficits exhibited seizures, and even post-mortem studies of patients with temporal lobe epilepsy found anomalies of these receptors. 16 Furthermore, changes in extracellular GABA levels have been reported in mice that had experienced seizures, but also in mice that explored a new space and in humans experiencing pain.17
GABA beyond inhibition
To further complicate matters, there are instances in which GABA does not inhibit the downstream neuron but, on the contrary, excites it. Time to get back down to technicalities for a second. As we have said, the primary way in which GABA inhibits a neuron is by allowing chlorine to flow in. That is, in normal circumstances, when chlorine is found at a low concentration inside the neuron. What GABA does is simply open the gate. Chlorine then flows according to its gradient, from the high concentration outside the cell towards the low concentration inside. 18
What if, for some reason, chlorine was abnormally high inside the neuron? GABA binds to its receptor, opens the gate, and chlorine follows the gradient – except now in a reverse direction, from inside out. This would obtain the opposite effect and excite (depolarize) the neuron. 18 GABA plays this excitatory role in very young neurons, during neurodevelopment, as well as in a particular subset of neurons in the pituitary.19,20
Targeting GABA
Interestingly enough, molecules that were explicitly designed to mimic GABA, such as Pregabalin 21 and Gabapentin22, seem to bind a calcium channel instead of the GABA receptor. Although they surprisingly do have anticonvulsant effects and may be used as anti-epileptics, they found their main role in pain management and other muscle-related diseases.
We have, however, managed to find other ways to target the GABA receptor and take advantage of its effects. Perhaps ‘found’ is a strong word. We first came across the effects, and later figured out the mechanisms.
In 1903, the barbituric acid derivative barbital was first synthesized and proven to induce sleep in dogs. Soon after, derivatives followed and were approved for use as sedatives, hypnotics, anti-epileptic drugs, and, starting with the 1930s, intravenous anesthetics. The drugs that changed the face of surgery act directly on the GABA-A receptor, although at a different site compared to GABA, prompting a generalized inhibition of the central nervous system. It wasn’t until the 1970s that the landmark studies of Macdonald and Barker showed the connection between barbiturates and GABA.23
Another class of drugs, benzodiazepines, was discovered in 1957 by chemist Leo Sternbach, who came across chlordiazepoxide rather by accident. This substance manifested unexpected sedative, anticonvulsant, and muscle-relaxing properties. Several analogs followed, like the classic Valium, which gained increasing popularity. It took 15 years for scientists to tie this widely prescribed medication to GABA.24 Benzodiazepines also act by directly binding the GABA-A receptor, at yet another site than GABA or barbiturates. Today, they still are among the most widely prescribed drugs, for a variety of issues such as anxiety, insomnia, epilepsy or alcohol withdrawal.25,26
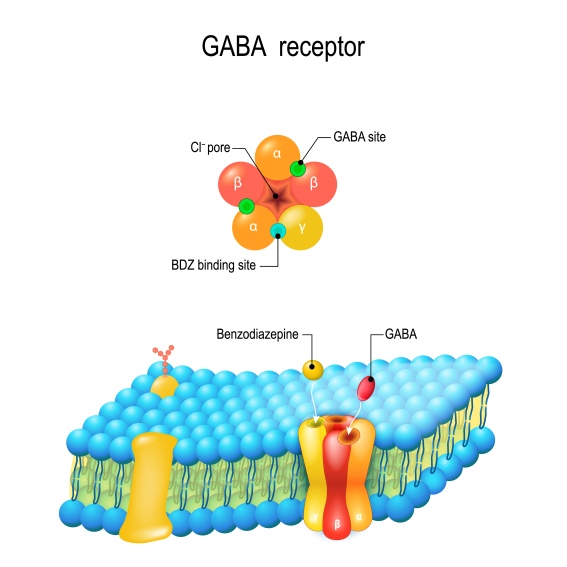
Oddly, benzodiazepines seem to fail their role in a most dire situation: status epilepticus, a life-threatening condition describing an epileptic seizure that lasts more than 5 minutes. During this particular state, GABA receptors appear to either be inactivated or internalized within the cell, often rendering benzodiazepines, along with other anti-epileptic medications, inefficient. 27
All in all, it is safe to say that an apparently straightforward matter, such as the supposedly well-known role GABA plays in the brain, becomes infinitely more intricate once we dissect it carefully enough. Fortunately, these ever-smaller dissections bring us closer and closer to an accurate, albeit very complex, picture of how our neurons work.
Resources:
- Spiering, M. J. The discovery of GABA in the brain. Journal of Biological Chemistry 293, 19159–19160 (2018).
- Roberts, E. & Frankel, S. r-AMINOBUTYRIC iiCID IN BRAIN: ITS FORMATION FROM GLUTAMIC ACID*.
- Bazemore, A. W., Elliott, K. A. C. & Florey, E. Isolation of Factor I. J. Neurochem. 1, 334–339 (1957).
- Kandel, E., Schwartz, J., Jessell, T., Siegelbaum, S. & Hudspeth, A. J. Principles of Neural Science. (Elsevier, 2012).
- Chebib, M. & Johnston, G. A. R. THE ‘ABC’ OF GABA RECEPTORS: A BRIEF REVIEW. Clin. Exp. Pharmacol. Physiol. 26, 937–940 (1999).
- Snodgrass, S. R. GABA and Epilepsy: Their Complex Relationship and the Evolution of Our Understanding. J. Child Neurol. 7, 77–86 (1992).
- Werner, F.-M. & Covenas, R. Classical Neurotransmitters and Neuropeptides Involved in Generalized Epilepsy: A Focus on Antiepileptic Drugs. Curr. Med. Chem. 18, 4933–4948 (2011).
- Vigabatrin - DrugBank. Available at: link. (Accessed: 19th April 2020)
- Valproic acid - DrugBank. Available at: link. (Accessed: 19th April 2020)
- Nuss, P. Anxiety disorders and GABA neurotransmission: A disturbance of modulation. Neuropsychiatr. Dis. Treat. 11, 165–175 (2015).
- Gottesmann, C. GABA mechanisms and sleep. Neuroscience 111, 231–239 (2002).
- Yu, X. et al. GABA and glutamate neurons in the VTA regulate sleep and wakefulness. Nat. Neurosci. 22, 106–119 (2019).
- Cuypers, K., Maes, C. & Swinnen, S. P. Aging and GABA. Aging 10, 1186–1187 (2018).
- Pauwels, L., Maes, C. & Swinnen, S. P. Aging, inhibition and GABA. Aging 10, 3645–3646 (2018).
- Farrant, M. & Nusser, Z. Variations on an inhibitory theme: Phasic and tonic activation of GABA A receptors. Nature Reviews Neuroscience 6, 215–229 (2005).
- Lee, V. & Maguire, J. The impact of tonic GABAA receptor-mediated inhibition on neuronal excitability varies across brain region and cell type. Frontiers in Neural Circuits 8, 3 (2014).
- Song, I., Savtchenko, L. & Semyanov, A. Tonic excitation or inhibition is set by GABAA conductance in hippocampal interneurons. Nat. Commun. 2, 1–10 (2011).
- GABA Receptor Physiology and Pharmacology - Basic Neurochemistry - NCBI Bookshelf. Available at: link. (Accessed: 19th April 2020)
- Ben-Ari, Y. Excitatory actions of GABA during development: The nature of the nurture. Nature Reviews Neuroscience 3, 728–739 (2002).
- Tomiko, S. A., Taraskevich, P. S. & Douglas, W. W. GABA acts directly on cells of pituitary pars intermedia to alter hormone output. Nature 301, 706–707 (1983).
- Pregabalin - DrugBank. Available at: link. (Accessed: 19th April 2020)
- Gabapentin - DrugBank. Available at: link. (Accessed: 19th April 2020)
- Löscher, W. & Rogawski, M. A. How theories evolved concerning the mechanism of action of barbiturates. Epilepsia 53, 12–25 (2012).
- Wick, J. Y. The history of benzodiazepines. Consultant Pharmacist 28, 538–548 (2013).
- Griffin, C. E., Kaye, A. M., Rivera Bueno, F. & Kaye, A. D. Benzodiazepine pharmacology and central nervous system-mediated effects. Ochsner J. 13, 214–223 (2013).
- Diazepam - DrugBank. Available at: link. (Accessed: 19th April 2020)
- GABA Receptors in Status Epilepticus | Epilepsy Foundation. Available at: link. (Accessed: 19th April 2020)